Hydrothermic Reduction of Rutile-Ilmenite Mineral Producing an Oxyhydride η-Ti2FeO0.2H2.8: Towards In-Situ Hydrogen Production and Storage
Keywords:
Hydrogen, carbo-hydrothermic, ethanol-water, rutile-ilmenite, graphene oxide, Hydrogen, hydrothermic, ethanol-water, rutile-ilmenite, graphene oxideAbstract
As an alternative to the physical storage of hydrogen as compressed gas or liquid hydrogen requiring high-pressure tanks and cryogenic temperatures, the material-based storage of hydrogen in solids involves hydrogen uptake and release from the surface of adsorbents or within interstitials of hydrides. We report a hydrothermic reduction of rutile-ilmenite mineral into hydrogen-rich fibrous products, η-Ti2FeO0.2H2.8, in an ethanol-water system at 120°C for 4 hrs. As part of a project to generate hydrogen from water-ethanol system using advanced catalysts containing graphene oxide (GO) as carbon source, a system of 62.5 μg graphene oxide per g of rutile-ilmenite mineral was employed in a concentration of 50 mg/mL of ethanol-water solution. As well as in the original mineral, XRD of thermal annealed mineral between 500 and 800°C showed no hydride or phase change in rutile-ilmenite. With hydrothermal treatment of GO/rutile-ilmenite (50 mg/mL) in ethanol-water (1:1 v/v) at 120°C, a hydrogen-rich ferrotitanium hydride phase was formed, and there was a change in morphology from plate-like and granular particles into fibrous structures. Like the release of hydrogen by its ‘carriers’ (e.g., CaH2, NH4BH4, NaBH4, NH3, formic acid), it is anticipated that hydrogen was generated from the ethanol-water system in-situ, which reduced the rutile-ilmenite mineral into a hydride. EDX results showed that the reduction affected specifically the oxides of Fe and aluminosilicates in the mineral. The study demonstrated a possibility of in-situ hydrogen generation and storage via low-temperature graphene oxide hydrothermic reduction of rutile-ilmenite mineral in an ethanol-water system.
References
Aubertin, F.; Gonser, U. and Campbell, S. (1984). Hydride formation by zirconium-iron alloys and by η-phase Zr4Fe2O0. 6. Journal of Physics F: Metal Physics, 14(9): 2213.
Avarmaa, K.; Klemettinen, L.; Taskinen, P.; Lindberg, D.; Pihlasalo, J.; Johto, H. and Jokilaakso, A. (2022). Utilization of Scrap Metals as Reductants for Improved Ni and Cu Recoveries in Copper Smelting. Journal of Sustainable Metallurgy, 8(4): 1915-1931.
Casas-Ledón, Y.; Arteaga-Perez, L. E.; Morales-Perez, M. C. and Peralta-Suárez, L. M. (2012). Thermodynamic analysis of the hydrogen production from ethanol: first and second laws approaches. International Scholarly Research Notices, 2012.
David, W. I.; Makepeace, J. W.; Callear, S. K.; Hunter, H. M.; Taylor, J. D.; Wood, T. J. and Jones, M. O. (2014). Hydrogen production from ammonia using sodium amide. Journal of the American Chemical Society, 136(38): 13082-13085.
Eklöf-Österberg, C.; Nedumkandathil, R.; Häussermann, U.; Jaworski, A.; Pell, A. J.; Tyagi, M.; Jalarvo, N. H.; Frick, B.; Faraone, A. and Karlsson, M. (2018). Dynamics of hydride ions in metal hydride-reduced BaTiO3 samples investigated with quasielastic neutron scattering. The Journal of Physical Chemistry C, 123(4): 2019-2030.
Fjellvåg, Ø. S.; Øygarden, V.; Sørby, M. H. and Sjåstad, A. O. (2019). Crystal structure of LaSr3Fe3O9 and its phase relation with LaSr3Fe3O10. Journal of solid state chemistry, 275: 56-62.
Gao, W.; Guo, J. and Chen, P. (2019). Hydrides, amides and imides mediated ammonia synthesis and decomposition. Chinese Journal of Chemistry, 37(5): 442-451.
Gultom, N. S.; Abdullah, H. and Kuo, D.-H. (2019). Effects of graphene oxide and sacrificial reagent for highly efficient hydrogen production with the costless Zn (O, S) photocatalyst. International Journal of Hydrogen Energy, 44(56): 29516-29528.
Habashi, F. (2016). Ilmenite for pigment and metal production. Interdisciplinary Journal of Chemistry, 1(1): 28-33.
Hanaor, D. A. and Sorrell, C. C. (2011). Review of the anatase to rutile phase transformation. Journal of Materials science, 46(4): 855-874.
Hsieh, C.-T. and Hsueh, J.-H. (2016). Electrochemical exfoliation of graphene sheets from a natural graphite flask in the presence of sulfate ions at different temperatures. RSC Advances, 6(69): 64826-64831.
Janssen, A.; Putnis, A.; Geisler, T. and Putnis, C. (2010). The experimental replacement of ilmenite by rutile in HCl solutions. Mineralogical Magazine, 74(4): 633-644.
Karlsson, T.; Forsgren, C. and Steenari, B.-M. (2018). Recovery of antimony: a laboratory study on the thermal decomposition and carbothermal reduction of Sb (III), Bi (III), Zn (II) oxides, and antimony compounds from metal oxide varistors. Journal of Sustainable Metallurgy, 4(2): 194-204.
Kim, S. Y.; Saqlain, S.; Cha, B. J.; Zhao, S.; Seo, H. O. and Kim, Y. D. (2020). Annealing temperature-dependent effects of Fe-Loading on the visible light-driven photocatalytic activity of rutile TiO2 nanoparticles and their applicability for air purification. Catalysts, 10(7): 739.
Kobayashi, Y.; Hernandez, O. J.; Sakaguchi, T.; Yajima, T.; Roisnel, T.; Tsujimoto, Y.; Morita, M.; Noda, Y.; Mogami, Y. and Kitada, A. (2012). An oxyhydride of BaTiO3 exhibiting hydride exchange and electronic conductivity. Nature materials, 11(6): 507-511.
Liu, M.; Zhou, L.; Luo, X.; Wan, C. and Xu, L. (2020). Recent advances in noble metal catalysts for hydrogen production from ammonia borane. Catalysts, 10(7): 788.
Long, F.; Yoo, Y.; Jo, C.; Seo, S. M.; Jeong, H.; Song, Y.; Jin, T. and Hu, Z. (2009). Phase transformation of η and σ phases in an experimental nickel-based superalloy. Journal of alloys and compounds, 478(1-2): 181-187.
Ma, M.; Wang, D.; Hu, X.; Jin, X. and Chen, G. Z. (2006). A direct electrochemical route from ilmenite to hydrogen‐storage ferrotitanium alloys. Chemistry–A European Journal, 12(19): 5075-5081.
Nakahira, A.; Kubo, T. and Numako, C. (2010). Formation mechanism of TiO2-derived titanate nanotubes prepared by the hydrothermal process. Inorganic chemistry, 49(13): 5845-5852.
Osinga, T.; Frommherz, U.; Steinfeld, A. and Wieckert, C. (2004). Experimental investigation of the solar carbothermic reduction of ZnO using a two-cavity solar reactor. J. Sol. Energy Eng., 126(1): 633-637.
Schwertmann, U. (1991). Solubility and dissolution of iron oxides. Plant and soil, 130(1): 1-25.
Simons, B. and Woermann, E. (1978). Iron titanium oxides in equilibrium with metallic iron. Contributions to Mineralogy and Petrology, 66(1): 81-89.
Simpraditpan, A.; Wirunmongkol, T.; Pavasupree, S. and Pecharapa, W. (2013). Simple hydrothermal preparation of nanofibers from a natural ilmenite mineral. Ceramics International, 39(3): 2497-2502.
Stioui, C.; Fruchart, D.; Rouault, A.; Fruchart, R.; Roudaut, E. and Rebiere, J. (1981). Absorption d'hydrogene par Ti4Fe2O et diverses phases M6O. Materials Research Bulletin, 16(7): 869-876.
Stioui, M.; Resnik, A.; Reshotko, M.; Grayevsky, A.; Shaltiel, D.; Kaplan, N. and Rupp, B. (1988). Nuclear magnetic resonance and magnetization studies in Ti4Fe2O0. 4Hx. Journal of the Less Common Metals, 141(2): 177-190.
Taguchi, K.; Shinozaki, K.; Okumura, H.; Michioka, C.; Yoshimura, K. and Ishihara, K. N. (2020). Discovery of Amorphous Iron Hydrides via Novel Quiescent Reaction in Aqueous Solution. Scientific Reports, 10(1): 1-9.
Takeshita, H. T.; Tanaka, H.; Kiyobayashi, T.; Takeichi, N. and Kuriyama, N. (2002). Hydrogenation characteristics of Ti2Ni and Ti4Ni2X (X= O, N, C). Journal of alloys and compounds, 330: 517-521.
Wieckert, C. and Steinfeld, A. (2002). Solar thermal reduction of ZnO using CH 4: ZnO and C: ZnO molar ratios less than 1. J. Sol. Energy Eng., 124(1): 55-62.
Wiesinger, G. and Hilscher, G. (1991). Chapter 6 Magnetism of hydrides Handbook of Magnetic Materials (Vol. 6, pp. 511-584): Elsevier.
Yang, J.; Jin, Z.; Wang, X.; Li, W.; Zhang, J.; Zhang, S.; Guo, X. and Zhang, Z. (2003). Study on composition, structure and formation process of nanotube Na 2 Ti 2 O 4 (OH) 2. Dalton Transactions(20): 3898-3901.
Zanaveskin, K.; Lukashev, R.; Makhin, M. and Zanaveskin, L. (2014). Hydrothermal preparation of porous materials from a rutile–quartz concentrate. Ceramics International, 40(10): 16577-16580.
Zavaliy, I.; Cerny, R.; Riabov, A. and Saldan, I. (2007). Crystal structure of the eta-Ti 3 ZrNi 2 O 0.5 suboxide and its Ti 3 ZrNi 2 O 0.5 D 5.7 deuteride. Moscow University Chemistry Bulletin, 48(1): 187-193.
Zhou, D.; Li, D.; Yuan, S. and Chen, Z. (2022). Recent Advances in Biomass-Based Photocatalytic H2 Production and Efficient Photocatalysts: A Review. Energy & Fuels, 36(18): 10721-10731.
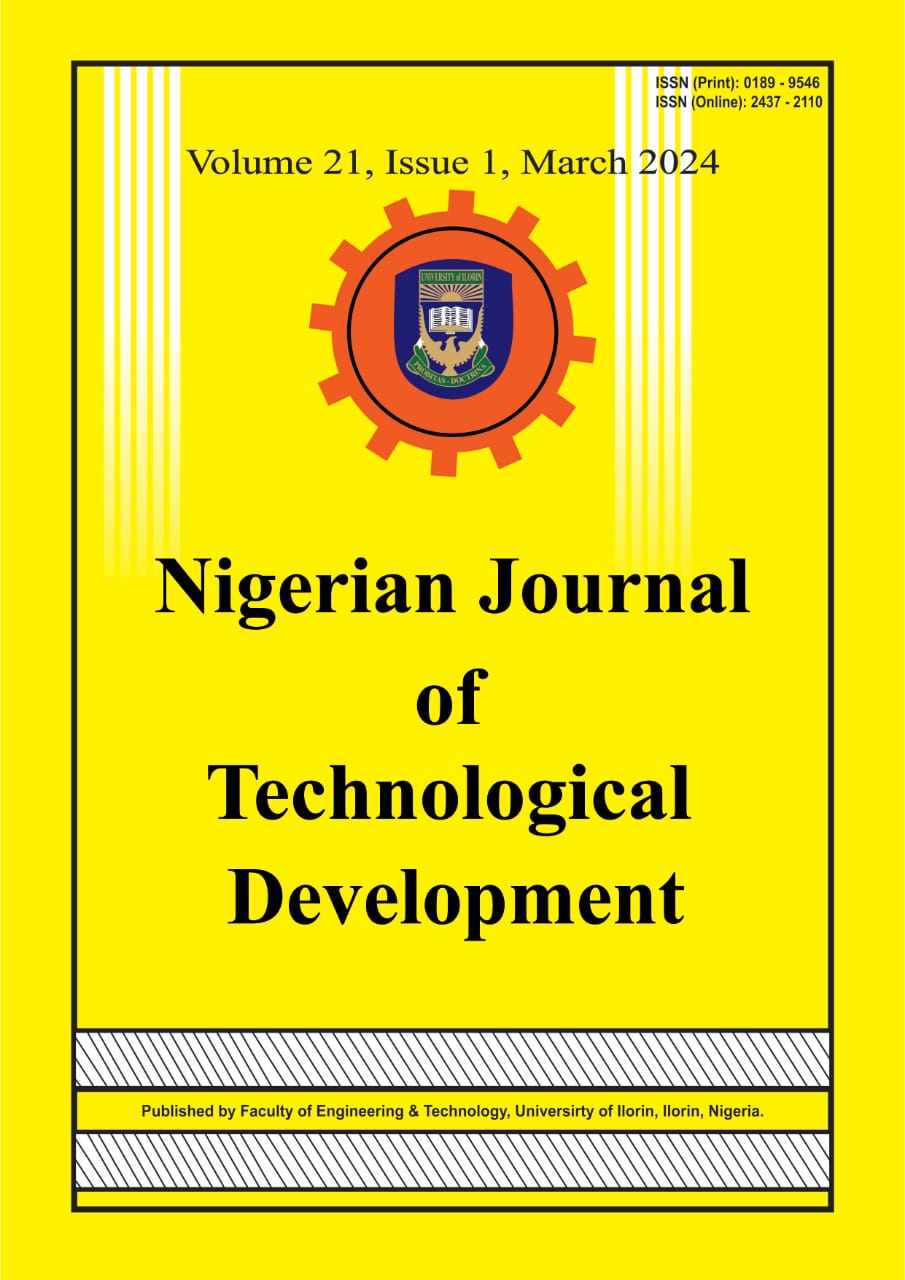
Downloads
Published
Issue
Section
License
Copyright (c) 2024 Nigerian Journal of Technological Development

This work is licensed under a Creative Commons Attribution-NonCommercial-ShareAlike 4.0 International License.
In accordance with the Copyright Act of 1976, which became effective January 1, 1978, the following statement signed by each author must accompany the manuscript submitted: "I, the undersigned author, transfer all copyright ownership of the manuscript referenced above to the Nigerian Journal of Technological Development, in the event the work is published. I warrant that the article is original, does not infringe upon any copyright or other proprietary right of any third party, is not under consideration by another journal, and has not been published previously. I have reviewed and approve the submitted version of the manuscript and agree to its publication in the Nigerian Journal of Technological Development." A copyright transfer form should be downloaded from the NJTD Website ( http://njtd.com.ng/index.php/njtd). Author(s) will be consulted, whenever possible, regarding republication of material. All authors must have access to the data presented and the authors and sponsor (if applicable) must agree to share original data with the editor if requested.