Evaluation of MgO-ZnO-Crab Shell Biofillers as Reinforcement for Biodegradable Polylactic Acid (PLA) Composite
Keywords:
Bone Fracture, degradation, hydroxyapatite, stability, hygroscopic characteristics, porosityAbstract
Biodegradable polyester obtained from renewable, eco-friendly materials, and natural additives made from debris of production of seafood to create biocomposites is nowadays a possibility. This paper evaluates the physical, morphological, and chemical properties and the degradation stability of polylactic acid/biofillers (magnesium oxide/zinc oxide/crab shell particles) composite as a viable biocomposite material in bone engineering applications. The biofiller showed hygroscopic characteristics. Surface morphology of the composite showed fractured surfaces with interconnected pores suitable for bone cells’ implantation enhancement and propagation. Biofillers effect accelerates the precipitation of calcium apatite formation after 28 days of immersion. The XRD spectra confirmed high composite crystallinity structure of 93.4% due to the nucleation effects of the biofillers. The beneficial role of reinforcing polylactic acid polymer with biofiller showed average pH value of 7.36 and apparent porosity of 40%. Findings from this paper have revealed that the use of crab shell debris such as crab shell can become a resource in biocomposite fabrication. The addition of biofillers provided an effective reinforcement in polylactic acid polymer matrix and hence contributed towards sustainable developments of natural resource materials and biodegradable and bioresorbable material without polluting the environment.
References
Aldana, A. A. and Abraham, G. A. (2017). Current advances in electrospun gelatin-based scaffolds for tissue engineering applications. International Journal of Pharmaceutics, 523(2), 441–453. https://doi.org/10.1016/j.ijpharm.2016.09.044
Antoniac, I.; M. Miculescu; V. Mănescu; A. Stere; P. H. Quan; G. Păltânea; A. Robu and K. Earar. (2022). Magnesium-based alloys used in orthopedic surgery. Materials, 15(3), 1148. https://doi.org/10.3390/ma15031148
Athanasoulia, I. G. I.; M. N. Christoforidis; D. M. Korres and P. A. Tarantili. (2017). The effect of hydroxyapatite nanoparticles on crystallization and thermomechanical properties of PLLA matrix. Pure and Applied Chemistry, 89(1), 125–140. https://doi.org/10.1515/pac-2016-0912
Bajpai, A.; J. Bajpai; R. K. Saini; P. Agrawal and A. Tiwari. (2016). Smart biomaterial devices: Polymers in biomedical sciences. CRC Press.
Balakrishnan, G.; R. Velavan; K. M. Batoo and E. H. Raslan. (2020). Microstructure, optical and photocatalytic properties of MgO nanoparticles. Results in Physics, 16, 103013. https://doi.org/10.1016/j.rinp.2020.103013
Dauda, K.; C. Majebi; A. Ayoola; O. Agboola; A. Popoola; O. Fayomi; S. Banjo; J. Sojobi and A. Ogunsanya. (2021). Biosmart materials and its innovative characteristics in protective composite coating application. 1107(1), 012216. https://doi.org/10.1088/1757-899X/1107/1/012216
Farshchi, N. and Ostad, Y. K. (2020). Sepiolite as a nanofiller to improve mechanical and thermal behavior of recycled high-density polyethylene. Progress in Rubber, Plastics and Recycling Technology, 36(3), 185–195. https://doi.org/10.1177/1477760620918596
Fattahi, F.; A. Khoddami and O. Avinc. (2019). Poly (lactic acid)(PLA) nanofibers for bone tissue engineering. Journal of Textiles and Polymers, 7(2), 47–64.
Funabashi, M.; F. Ninomiya; E. D. Flores and M. Kunioka. (2010). Biomass carbon ratio of polymer composites measured by accelerator mass spectrometry. Journal of Polymers and the Environment, 18, 85–93. https://doi.org/10.1007/s10924-010-0166-3
Gao, C.; S. Peng; P. Feng and C. Shuai (2017). Bone biomaterials and interactions with stem cells. Bone Research, 5(1), 1–33. https://doi.org/10.1038/boneres.2017.59
Gigante, V.; P. Cinelli; M. C. Righetti; M. Sandroni; L. Tognotti; M. Seggiani and A. Lazzeri. (2020). Evaluation of mussel shells powder as reinforcement for PLA-based biocomposites. International Journal of Molecular Sciences, 21(15), 5364. https://doi.org/10.3390/ijms21155364
Gortsas, T. V.; S. Tsinopoulos; E. Polyzos; L. Pyl; D. Fotiadis and D. Polyzos. (2022). BEM evaluation of surface octahedral strains and internal strain gradients in 3D-printed scaffolds used for bone tissue regeneration. Journal of the Mechanical Behavior of Biomedical Materials, 125, 104919. https://doi.org/10.1016/j.jmbbm.2021.104919
Gritsch, L.; M. Maqbool; V. Mouriño; F. E. Ciraldo; M. Cresswell; P. R. Jackson; C. Lovell and A. R. Boccaccini. (2019). Chitosan/hydroxyapatite composite bone tissue engineering scaffolds with dual and decoupled therapeutic ion delivery: Copper and strontium. Journal of Materials Chemistry B, 7(40), 6109–6124. https://doi.org/10.1039/C9TB00897G
Hamester, M. R. R.; P. S. Balzer and D. Becker (2012). Characterization of calcium carbonate obtained from oyster and mussel shells and incorporation in polypropylene. Materials Research, 15(2), 204–208. https://doi.org/10.1590/S1516-14392012005000014
Iqbal, N.; T. M. Braxton; A. Anastasiou; E. M. Raif; C. K. Y. Chung; S. Kumar; P. V. Giannoudis and A. Jha. (2022). Dicalcium Phosphate Dihydrate Mineral Loaded Freeze-Dried Scaffolds for Potential Synthetic Bone Applications. Materials, 15(18), 6245. https://doi.org/10.3390/ma15186245
Jahani, B.; K. Meesterb; X. Wanga and A. Brooksc. (2020). Biodegradable Magnesium-Based alloys for bone repair applications: Prospects and challenges. Biomed Sci Instrum, 56(2), 292–304.
Jayaramudu, J.; K. Das; M. Sonakshi; G. S. M. Reddy; B. Aderibigbe; R. Sadiku and S. S. Ray. (2014). Structure and properties of highly toughened biodegradable polylactide/ZnO biocomposite films. International Journal of Biological Macromolecules, 64, 428–434. https://doi.org/10.1016/j.ijbiomac.2013.12.034
Kim, I.; K. Viswanathan; G. Kasi; K. Sadeghi; S. Thanakkasaranee and J. Seo. (2019). Poly (lactic acid)/ZnO bionanocomposite films with positively charged ZnO as potential antimicrobial food packaging materials. Polymers, 11(9), 1427. https://doi.org/10.3390/polym11091427
Kunitake, M. E.; L. M. Mangano; J. M. Peloquin; S. P. Baker and L. A. Estroff. (2013). Evaluation of strengthening mechanisms in calcite single crystals from mollusk shells. Acta Biomaterialia, 9(2), 5353–5359. https://doi.org/10.1016/j.actbio.2012.09.030
Levengood, S. K. L. and Zhang, M. (2014). Chitosan-based scaffolds for bone tissue engineering. Journal of Materials Chemistry B, 2(21), 3161–3184. https://doi.org/DOI https://doi.org/10.1039/C4TB00027G
Li, H. Y.; Y. Q. Tan; L. Zhang; Y. X. Zhang; Y. H. Song; Y. Ye and M. S. Xia. (2012). Bio-filler from waste shellfish shell: Preparation, characterization, and its effect on the mechanical properties on polypropylene composites. Journal of Hazardous Materials, 217–218, 256–262. https://doi.org/10.1016/j.jhazmat.2012.03.028
Li, X.; W. Yu; L. Han; C. Chu; J. Bai and F. Xue (2019). Degradation behaviors of Mg alloy wires/PLA composite in the consistent and staged dynamic environments. Materials Science and Engineering: C, 103, 109765. https://doi.org/10.1016/j.msec.2019.109765
Monia, T. (2022). β-TCP/DCPD-PHBV (40%/60%): Biomaterial made from bioceramic and biopolymer for bone regeneration; investigation of intrinsic properties. Journal of Applied Biomaterials & Functional Materials, 20, 22808000221088950. https://doi.org/10.1177/22808000221088950
Morsi, M. A. and Abd Elhamid, M. H. (2019). Effect of iron doped hydroxyapatite nanoparticles on the structural, morphological, mechanical and magnetic properties of polylactic acid polymer. Journal of Materials Research and Technology, 8(2), 2098–2106. https://doi.org/10.1016/j.jmrt.2019.01.017
Offner, D.; G. F. de Grado; I. Meisels; L. Pijnenburg; F. Fioretti; N. Benkirane-Jessel and A. M. Musset. (2019). Bone grafts, bone substitutes and regenerative medicine acceptance for the management of bone defects among French population: Issues about ethics, religion or fear? Cell Medicine, 11, 2155179019857661. https://doi.org/10.1177/2155179019857661
Palaniyappan, S. and kumar Sivakumar, N. (2023). Development of crab shell particle reinforced polylactic acid filaments for 3D printing application. Materials Letters, 341, 134257. https://doi.org/10.1016/j.matlet.2023.134257
Phetwarotai, W. and Aht-Ong, D. (2017). Nucleated polylactide blend films with nanoprecipitated calcium carbonate and talc: Preparation, properties, and crystallization kinetics. Journal of Thermal Analysis and Calorimetry, 127, 2367–2381. https://doi.org/10.1007/s10973-016-5802-2
Qu, H.; H. Fu; Z. Han and Y. Sun. (2019). Biomaterials for bone tissue engineering scaffolds: A review. RSC Advances, 9(45), 26252–26262. https://doi.org/10.1039/c9ra05214c
Radwan-Pragłowska, J.; Ł. Janus; M. Piątkowski; D. Bogdał and D. Matysek. (2020). 3D hierarchical, nanostructured chitosan/PLA/HA scaffolds doped with TiO2/Au/Pt NPs with tunable properties for guided bone tissue engineering. Polymers, 12(4), 792. https://doi.org/10.3390/polym12040792
Rajkumar, K.; P. Sirisha and M. R. Sankar. (2014). Tribomechanical and surface topographical investigations of poly methyl methacrylate-seashell particle based biocomposite. Procedia Materials Science, 5, 1248–1257. https://doi.org/10.1016/j.mspro.2014.07.436
Reina, S.; B. Tito; M. Malini; F. Iqrimatien and E. Sa’diyah. (2021). Porosity and compressive strength of PLA-based scaffold coated with hydroxyapatite-gelatin to reconstruct mandibula: A literature review. 1816(1), 012085. https://doi.org/10.1088/1742-6596/1816/1/012085
Saravanan, S.; S. Vimalraj; G. Lakshmanan; A. Jindal; D. Sundaramurthi and J. Bhattacharya. (2019). Chitosan-based biocomposite scaffolds and hydrogels for bone tissue regeneration. Marine-Derived Biomaterials for Tissue Engineering Applications, 413–442.
Sarki, J.; S. Hassan; V. Aigbodion and J. Oghenevweta. (2011). Potential of using coconut shell particle fillers in eco-composite materials. Journal of Alloys and Compounds, 509(5), 2381–2385. https://doi.org/10.1016/j.jallcom.2010.11.025
Shi, J. (2016). Development of functionally graded implant materials in commercial use. Available online at: https://mpra.ub.uni-muenchen.de/id/eprint/76351. Accessed on March 7, 2023.Shi, X.; G. Zhang; T. V. Phuong and A. Lazzeri. (2015). Synergistic effects of nucleating agents and plasticizers on the crystallization behavior of poly (lactic acid). Molecules, 20(1), 1579–1593. https://doi.org/10.3390/molecules20011579
Swaroop, C. and Shukla, M. (2018). Nano-magnesium oxide reinforced polylactic acid biofilms for food packaging applications. International Journal of Biological Macromolecules, 113, 729–736. https://doi.org/10.1016/j.ijbiomac.2018.02.156
Ting, X.; Y. Hongyang; Y. Dongzhi and Y. Zhong-Zhen. (2017). Polylactic Acid Nanofiber Scaffold Decorated with Chitosan Islandlike Topography for Bone Tissue Engineering. 9(25), 21094–21104. https://doi.org/10.1021/acsami.7b01176
Trimeche, M. (2017). Biomaterials for bone regeneration: An overview. Biomater. Tissue Technol, 1, 1–5.
Wang, Z.; Y. Tang; M. Yakufu; L. Li; G. Li; J. Liu and P. Zhang. (2020). Highly Permeable Gelatin/Poly (lactic acid) Fibrous Scaffolds with a Three-Dimensional Spatial Structure for Efficient Cell Infiltration, Mineralization and Bone Regeneration. ACS Applied Bio Materials, 3(10), 6932–6943. https://doi.org/10.1021/acsabm.0c00815
Witte, F. (2010). The history of biodegradable magnesium implants: A review. Acta Biomaterialia, 6(5), 1680–1692. https://doi.org/10.1016/j.actbio.2010.02.028
Yang, F.; X. Ye; J. Zhong; Z. Lin; S. Wu; Y. Hu; W. Zheng; W. Zhou; Y. Wei and X. Dong. (2023). Recycling of waste crab shells into reinforced poly (lactic acid) biocomposites for 3D printing. International Journal of Biological Macromolecules, 234, 122974. https://doi.org/10.1016/j.ijbiomac.2022.12.193
Yasmeen, S.; M. K. Kabiraz; B. Saha; M. Qadir; M. Gafur and S. Masum. (2016). Chromium (VI) ions removal from tannery effluent using chitosan-microcrystalline cellulosecomposite as adsorbent. Int. Res. J. Pure Appl. Chem, 10(4), 1–14. https://doi.org/10.9734/IRJPAC/2016/23315
Zhao, Y.; H. Liang; S. Zhang; S. Qu; Y. Jiang and M. Chen. (2020). Effects of magnesium oxide (MgO) shapes on in vitro and in vivo degradation behaviors of PLA/MgO composites in long term. Polymers, 12(5), 1074. https://doi.org/10.3390/polym12051074.
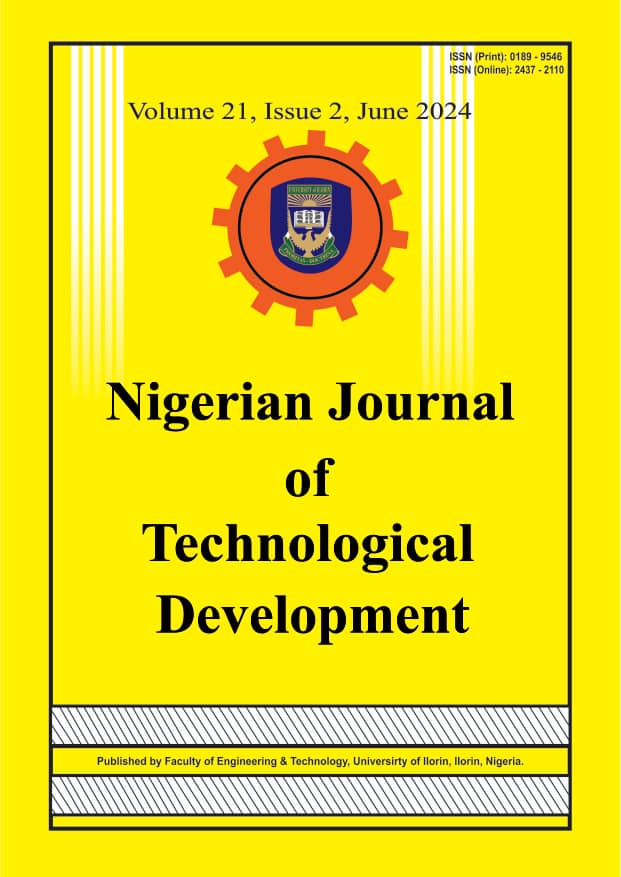
Downloads
Published
Issue
Section
License
Copyright (c) 2024 Nigerian Journal of Technological Development

This work is licensed under a Creative Commons Attribution-NonCommercial-ShareAlike 4.0 International License.
In accordance with the Copyright Act of 1976, which became effective January 1, 1978, the following statement signed by each author must accompany the manuscript submitted: "I, the undersigned author, transfer all copyright ownership of the manuscript referenced above to the Nigerian Journal of Technological Development, in the event the work is published. I warrant that the article is original, does not infringe upon any copyright or other proprietary right of any third party, is not under consideration by another journal, and has not been published previously. I have reviewed and approve the submitted version of the manuscript and agree to its publication in the Nigerian Journal of Technological Development." A copyright transfer form should be downloaded from the NJTD Website ( http://njtd.com.ng/index.php/njtd). Author(s) will be consulted, whenever possible, regarding republication of material. All authors must have access to the data presented and the authors and sponsor (if applicable) must agree to share original data with the editor if requested.