Biomechanical and Physical Properties Selection of Ti-Ha-CaCO3 Biocomposite Prostheses for Replacement of Bone Atrophy
Keywords:
Biocomposite, Biomechanical, Structure, Properties, Prosthesis and Image J, Biocomposite, Biomechanical, Structure, Properties, Prosthesis, ImageJAbstract
Traditional prosthetic materials often lack the desired properties to mimic the mechanical behaviour of natural bone, leading to complications and reduced implant longevity. This study aims to conduct a biomechanical and physical properties selection analysis for biocomposite prostheses' suitable for replacing bone atrophy. This involves evaluating the mechanical properties of developed biocomposites with different structures (dense, porous and gradient) to ensure compatibility with the mechanical properties of bone. The radar chart was adopted to compare and evaluate the mechanical strength of various biocomposite implants and identify the most suitable prosthesis for load-bearing bone replacement. The study utilises powder metallurgy, scanning electron microscopy (SEM), and ImageJ software to produce and characterise the pore size distribution of the biocomposites, respectively. The findings of this study revealed the gradient and porous biocomposites exhibited desired mechanical properties with porosity of 20.67 and 27.72 % pore size up to 134 and 256 μm, compressive strength of 174 and 149.29 MPa and compressive modulus of 30.42 and 28.3 GPa respectively. The SEM analysis, coupled with pore size distribution and porosity percentage measurements, offers valuable information for designing and fabricating biomaterials with enhanced properties. The gradient biocomposite was identified to be the best sample for load-bearing bone replacements by the selection analysis because of its high compressive strength and low modulus, which is within the established cortical bone mechanical properties.
References
Abdel Wahab, A. A. and Silberschmidt, V. V. (2011). Numerical Modelling Of Impact Fracture Of Cortical Bone Tissue Using X-Fem. Journal Of Theoretical And Applied Mechanics, 49, 3, pp. 599-619, Warsaw 2011.
Abutu, J.; S. A. Lawal; M. B. Ndaliman; R. A. Lafia-Araga; O. Adedipe and I. A. Choudhury. (2018). Effects of process parameters on the properties of brake pad developed from seashell as reinforcement material using grey relational
analysis. Engineering Science and Technology, an International Journal, 21(4), 787–797. doi:10.1016/j.jestch.2018.05.014
Adoba, A. E.;H. B. McShane; R. D. Rawlings and I. U. Rehman. (1997). Study of the Mechanical Properties and Bioactivity of Functionally Graded Titanium Matrix Composites Reinforced with Bioactive Particles. In Proceeding: Eleventh International Conference on Composite Materials, Australian Composite Structures Society Woodhead Publishing Limited, Queensland,
Australia.
Afzal M. A. F.; P. Kesarwani and K. M. Reddy. (2012). Functionally graded hydroxyapatite-alumina-zirconia biocomposite: synergy of toughness and biocompatibility. Mat Sci Eng C 2012; 32(5): 1164–1173
Akoh, C.C.; J. Chen; R. Kadakia; Y.U. Park; H. Kim and S.B. Adams. (2021). Adverse events involving hallux metatarsophalangeal joint implants: analysis of the United States Food and Drug Administration data from 2010 to 2018. Foot and Ankle Surgery, 27(4), pp.381-388.
Arif, S.; M. Alam; A.H. Ansari; M.A. Siddiqui and M. Mohsin. (2017). Study of mechanical and tribological behaviour of Al/SiC/ZrO2 hybrid composites fabricated through powder metallurgy technique, Mater. Res. Express 4, 07651, https://doi.org/10.1088/Mater. Res. Express 4 (2017) 076511 2053-1591/aa7b5f.
Arifin, A.; A. B. Sulong; A. S. Mohruni and I. Yani. (2014). Palm stearin as alternative binder for MIM: a review. J Ocean, Mech Aerosp Sci Eng, 7, 18-23.
Arifin, A.; A. B. Sulong; L. C. Fun; Gunawan and Yani, I. (2017). Porous titanium alloy/hydroxyapatite composite using powder compaction route. Journal of Mechanical Engineering and Sciences, Volume 11, Issue 2, pp. 2679-2692, DOI: https://doi.org/10.15282/jmes.11.2.2017.10.0244
Bahraminasab, M. and Farahmand, F. (2017). State of the art review on design and manufacture of hybrid biomedical materials: Hip and knee prostheses. ProcIMechE Part H: J Engineering in Medicine 1–29. DOI: 10.1177/0954411917705911.
Balbinotti, P.; E. Gemelli; G. Buerger; S. A. D. Lima; J. D. Jesus; N. H. A. Camargo ... and Soares, G. D. D. A. (2011). Microstructure development on sintered Ti/HA biocomposites produced by powder metallurgy. Materials Research, 14, 384-393.
Barua, E.; P. Deb; S. Das Lala and A. B. Deoghare. (2019). Extraction of hydroxyapatite from bovine bone for sustainable development. Biomaterials in Orthopaedics and Bone Regeneration: Design and Synthesis, 147-158.
Batin, G.; C. Popa; L. Brânduşan and I. Vida-Simiti. (2011). Mechanical Properties Of Ti/Ha Functionally Graded Materials For Hard Tissue Replacement. Powder Metallurgy Progress, Vol.11 (2011), No 3-4, pp. 206-209
Cabezas-Villa, J. L.; L. Olmos; D. Bouvard; J. Lemus-Ruiz and O. Jiménez. (2018). Processing and properties of highly porous Ti6Al4V mimicking human bones. Journal of Materials Research, 33(06), 650–661. doi:10.1557/jmr.2018.35
Cetinel, O.; Z. Esen and B. Yildirim. (2019). Fabrication, Morphology Analysis, and Mechanical Properties of Ti Foams Manufactured Using the Space Holder Method for Bone Substitute Materials. Metals, 9(3), 340. doi:10.3390/met9030340
Choy, M. T.; C.-Y. Tang; L. Chen; W.-C. Law; C.-P. Tsui and W. W. Lu. (2015). Microwave assisted-in situ synthesis of porous titanium/calcium phosphate composites and their in vitro apatite-forming capability. Composites Part B: Engineering, 83, 50–57. doi:10.1016/j.compositesb.2015.08.04
El-Hajje, A.; E. C. Kolos; J. K. Wang; S. Maleksaeedi; Z. He; F. E. Wiria; C. Choong and A. J. Ruys. (2014). Physical and mechanical characterisation of 3D-printed porous titanium for biomedical applications. Journal of Materials Science: Materials in Medicine, 25(11), 2471–2480. doi:10.1007/s10856-014-5277-2.
Hamandi, F.; J.T. Tsatalis and T. Goswami. (2022). Retrospective Evaluation and Framework Development of Bone Anisotropic Material Behavior Compared with Elastic, Elastic-Plastic, and Hyper-Elastic Properties. Bioengineering 2022, 9, 9. https://doi.org/10.3390/ bioengineering9010009
Holota, T.; M. Kotus; M. Holienčinová; J. Mareček and M. Zach. (2015). Application of radar chart in the selection of material for clutch plates. Acta Universitatis Agriculturae et Silviculturae Mendelianae Brunensis, 63(1), 39-43.
Jawad K. O.; A. A. Rana and A. M. Sura. (2015). Fabrication, characterisation and Physical Properties of Functionally Graded Ti/HAP bioimplants, Wulfenia Journal Vol 22, No. 7 pp. 336-348.
Jelitto, H. and Schneider, G. A. (2019). Fracture toughness of porous materials – Experimental methods and data. Data in Brief, 103709. doi:10.1016/j.dib.2019.103709
Jeong, W.; S.-E. Shin; and H. Choi. (2020). Microstructure and Mechanical Properties of Titanium–Equine Bone Biocomposites. Metals, 10(5), 581. doi:10.3390/met10050581
Kaivosoja, E.; V.-M. Tiainen; Y. Takakubo; B. Rajchel; J. Sobiecki; Y. T. Konttinen and V. Takagi. (2013). Materials used for hip and knee implants. Wear of Orthopaedic Implants and Artificial Joints, 178–218. doi:10.1533/9780857096128.1.178
Krishna, E. S. and Suresh, G. (2022). Bioactive Titanium-Hydroxyapatite Composites by Powder Metallurgy Route, Volume 12, Issue 4, 2022, 5375 – 5383 https://doi.org/10.33263/BRIAC124.53755383
Li, C. and Zhou, Z. (2021). Charpy Impact Behavior of a Novel Stainless Steel Powder Wire Mesh Composite Porous Plate. Materials, 14, 2924. https://doi.org/10.3390/ma14112924
Mara, F. C. V. T. (2015). Mechanical Characterisation of Porous Titanium Implant Materials, PhD thesis, Universidade do Minho, Escola de Engenharia.
Matuła, I.; G. Dercz; M. Sowa; A. Barylski and P. Duda. (2021). Fabrication and Characterisation of New Functional Graded Material Based on Ti, Ta, and Zr by Powder Metallurgy Method. Materials, 14, 6609. https://doi.org/10.3390/ma14216609.
Moghadasi, K., Isa, M. S. M., Ariffin, M. A., Mohd jamil, M. Z., Raja, S., Wu, B., Yamani, M., Muhamad, M. R., Yusof, F., Jamaludin, M. F., Ab Karim, M. S., Abdul Razak, B. and Yusoff, N. (2022). A review on biomedical implant materials and the effect of friction stir-based techniques on their mechanical and tribological properties, Journal of Materials Research and Technology, Volume 17, pp. 1054-1121, https://doi.org/10.1016/j.jmrt.2022.01.050.
Morgan, E. F., Unnikrisnan, G. U. and Hussein, A. I. (2018). Bone Mechanical Properties in Healthy and Diseased States. Annual Review of Biomedical Engineering, 20(1), 119–143. doi:10.1146/annurev-bioeng-062117-121139
Nawawi, N. A., Alqap, A. S. F. and Sopyan, I (2011). Recent Progress on Hydroxyapatite-Based Dense Biomaterials for Load Bearing Bone Substitutes, Recent Patients on Materials Science, Volume 4, pp. 63-80.
Niespodziana, K. (2019). Synthesis and properties of porous Ti-20 wt.% HA nanocomposites. Journal of Materials Engineering and Performance, 28(4), 2245-2255.
Nurul Amin, A. K. M., Nasir, S. N. M., Khalid, N. S. and Arif, M. D. (2012). Comparison of Surface Roughness Attainable in High Speed End Milling of Silicon with Air Blowing on Conventional and CNC Milling Machines. Advanced Materials Research, 576, 1922. doi:10.4028/www.scientific.net/amr.576.19.
Oliveira, T. J. A.; F. A. M. Cássaro and L. F. Pires. (2020). The porous size distribution obtained and analysed by free access software. Revista Brasileira de Ensino de Física, vol. 42, e20200192: 1-4, DOI: https://doi.org/10.1590/1806-9126-RBEF-20200192.
Oshkour, A. A.; S. Pramanik; M. Mehrali; Y. H. Yau; F. Tarlochan and N. A. Abu Osman. (2015). Mechanical and physical behavior of newly developed functionally graded materials and composites of stainless steel 316L with calcium silicate and hydroxyapatite. Journal of the Mechanical Behavior of Biomedical Materials, 49, 321–331. doi:10.1016/j.jmbbm.2015.05.020
Petersen, R. (2014). Titanium Implant Osseointegration Problems with Alternate Solutions Using Epoxy/Carbon-Fiber-Reinforced Composite. Metals, 4(4), 549–569. doi:10.3390/met4040549
Poliakov A.; V. Pakhaliuk and V. L. Popov. (2019). Current Trends in Improving of Artificial Joints Design and Technologies for Their Arthroplasty. Front. Mech. Eng. 6:4. doi:10.3389/fmech.2020.00004.
Porter, M. M. and Niksiar, P. (2018). Multidimensional mechanics: Performance mapping of natural biological systems using permutated radar charts. PloS one, 13(9), e0204309.
Prakash, K. S.; P. M. Gopal; D. Anburose and V. Kavimani. (2016). Mechanical, corrosion and wear characteristics of powder metallurgy processed Ti-6Al-4V/B4C metal matrix composites. Ain Shams Engineering Journal. doi:10.1016/j.asej.2016.11.003
Prakash, C.; S. Singh; S. Ramakrishna; G. Królczyk and C. H. Le. (2020). Microwave sintering of porous Ti–Nb-HA composite with high strength and enhanced bioactivity for implant applications. Journal of Alloys and Compounds, 824, 153774.
Prasad, K.; O. Bazaka; M. Chua; M. Rochford; L. Fedrick; J. Spoor; … and Bazaka, K. (2017). Metallic Biomaterials: Current Challenges and Opportunities. Materials, 10(8), 884. doi:10.3390/ma10080884.
Qian, C.; F. Zhang and J. Sun. (2015). Fabrication of Ti/HA composite and functionally graded implant by three-dimensional printing. Bio-Medical Materials and Engineering, 25(2), 127–136. doi:10.3233/bme-151263
Saba, F.; F. Zhang; S. Liu and T. Liu. (2018). Reinforcement size dependence of mechanical properties and strengthening mechanisms in diamond reinforced titanium metal matrix composites. Composites Part B: Engineering. doi:10.1016/j.compositesb.2018.12.014.
Safari, H.; B. J. Balcom and A. Afrough. (2021). Characterisation of pore and grain size distributions in porous geological samples–An image processing workflow. Computers & Geosciences, 156, 104895.
Saraf, S.; A. Singh and B. G. Desai. (2019). Estimation of Porosity and Pore size distribution from Scanning Electron Microscope image data of Shale Samples: A Case Study on Jhuran Formation of Kachchh Basin, India. ASEG Extended Abstracts, 2019(1), 1–3. doi:10.1080/22020586.2019.12073197.
Sedighi, M.; N. Omidi and A. H. Jabbari. (2017). Experimental Investigation of FGM Dental Implant Properties Made from Ti/HA Composite, Mechanics of Advanced Composite Structures 4 (2017) 233-237.
Saxena, V.; V. Kumar; A. Rai; R. Yadav; U. Gupta; V. K. Singh and P. P. Manna. (2019). Optimisation of the bio-mechanical properties of Ti–8Si–2Mn alloy by 1393B3 bioactive glass reinforcement. Materials Research Express, 6(7), 075401.
Shahrjerdi, A.; F. Mustapha; M. Bayat; S. M. Sapuan and D. L. A. Majid. (2011). Fabrication of functionally graded HydroxyapatiteTitanium by applying optimal sintering procedure and powder metallurgy. International Journal of the Physical Sciences Vol. 6(9), pp. 2258-2267,DOI: 10.5897/IJPS11.223.
Sikora-Jasinska, M.; C. Paternoster; E. Mostaed; R. Tolouei; R. Casati; M. Vedani and D. Mantovani. (2017). Synthesis, mechanical properties and corrosion behavior of powder metallurgy processed Fe/Mg2Si composites for biodegradable implant applications, Materials Science and Engineering C, doi: 10.1016/j.msec.2017.07.049.
Soro, N.; L. Brassart; Y. Chen; M. Veidt; H. Attar and M. S. Dargusch. (2018). Finite element analysis of porous commercially pure titanium for biomedical implant application. Materials Science and Engineering: A, 725, 43–50. doi:10.1016/j.msea.2018.04.009
Taniguchi, N.; S. Fujibayashi; M.Takemoto; K. Sasaki; B. Otsuki; T. Nakamura; T. Matsushita; T. Kokubo and S. Matsuda. (2016). Effect of pore size on bone ingrowth into porous titanium implants fabricated by additive manufacturing: An in vivo experiment. Materials Science and Engineering: C, 59, 690–701. doi:10.1016/j.msec.2015.10.069
Tharaknath, S.; R. Ramkumar and B. Lokesh. (2016). Middle-East Journal of Scientific Research 24 (Recent Innovations in Engineering, Technology, Management & Applications): 124-132, DOI: 10.5829/idosi.mejsr.2016.24.RIETMA120.
Wan, Y.; K. Cheng; Z. Liu and H. Ye. (2013). An investigation on machinability assessment of difficult-to-cut materials based on radar charts. Proceedings of the Institution of Mechanical Engineers, Part B: Journal of Engineering Manufacture, 227(12), 1916-1920.
Wo, J.; S. Huang; D. Wu; J. Zhu; Z. Li and F. Yuan. (2020). The integration of pore size and porosity distribution on Ti-6A1-4V scaffolds by 3D printing in the modulation of osteo-differentation, Journal of Applied Biomaterials & Functional Materials, Volume 18: 1–10, DOI: 10.1177/2280800020934652
Zakaria, M.Y.; A.B. Sulong; M.I. Ramli; B. Ukwueze; W.S.W. Harun and R.L. Mahmun. (2018). Fabrication of Porous Titanium hydroxyapatite Composite Via Powder Metallurgy With Space Holder Method Journal of Advanced Manufacturing Technology (JAMT), Vol. 12 No. 1, pp. 37-48.
Zakaria, M. Y.; M. I. Ramli; A. B. Sulong; N Muhamad and M. H. Ismail. (2021). Application of sodium chloride as space holder for powder injection molding of alloy Titanium–Hydroxyapatite composites. Journal of Materials Research and Technology, 12, 478–486. doi:10.1016/j.jmrt.2021.02.087.
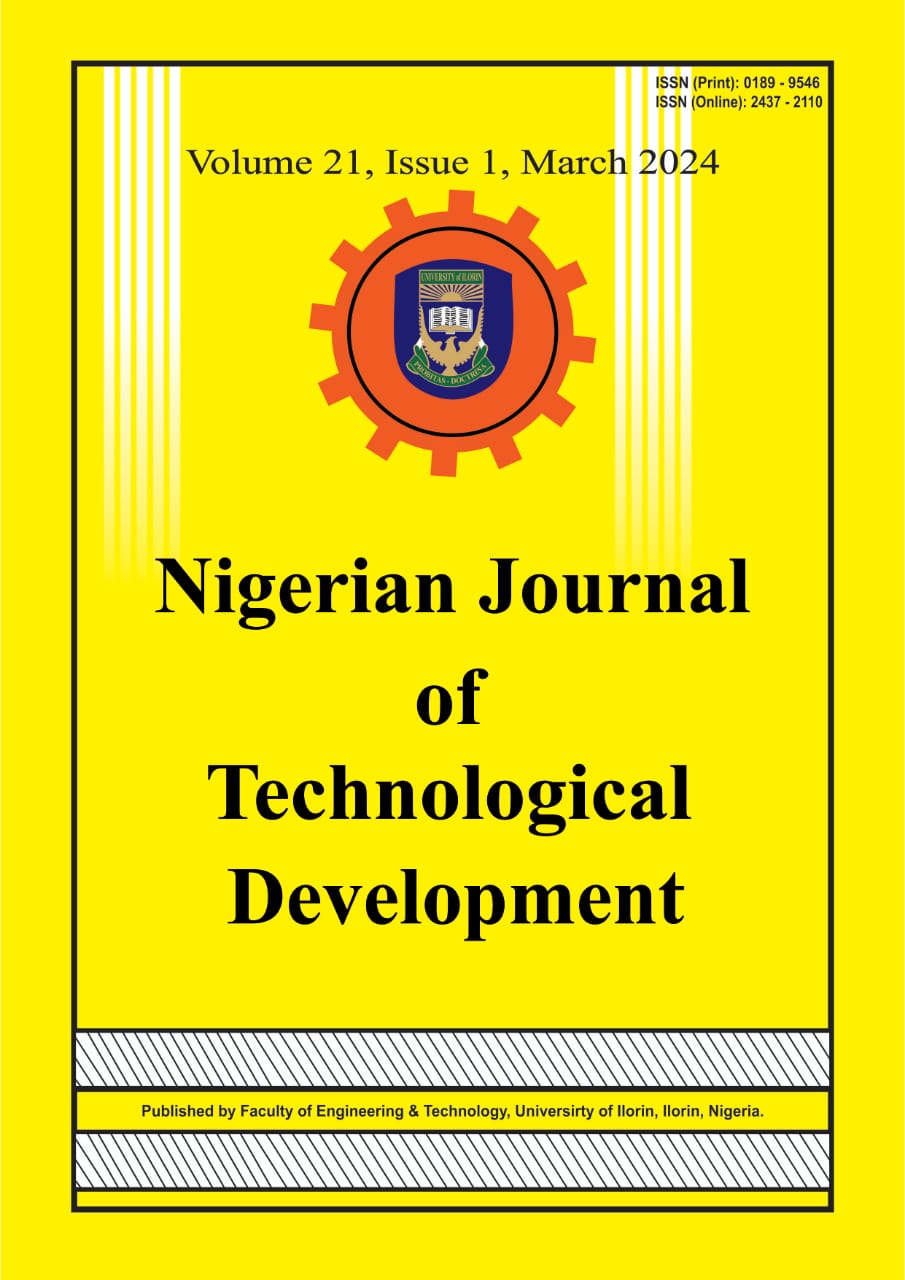
Downloads
Published
Issue
Section
License
Copyright (c) 2024 Nigerian Journal of Technological Development

This work is licensed under a Creative Commons Attribution-NonCommercial-ShareAlike 4.0 International License.
In accordance with the Copyright Act of 1976, which became effective January 1, 1978, the following statement signed by each author must accompany the manuscript submitted: "I, the undersigned author, transfer all copyright ownership of the manuscript referenced above to the Nigerian Journal of Technological Development, in the event the work is published. I warrant that the article is original, does not infringe upon any copyright or other proprietary right of any third party, is not under consideration by another journal, and has not been published previously. I have reviewed and approve the submitted version of the manuscript and agree to its publication in the Nigerian Journal of Technological Development." A copyright transfer form should be downloaded from the NJTD Website ( http://njtd.com.ng/index.php/njtd). Author(s) will be consulted, whenever possible, regarding republication of material. All authors must have access to the data presented and the authors and sponsor (if applicable) must agree to share original data with the editor if requested.